Original: https://link.springer.com/article/10.1007/s10126-008-9171-0
Marine Biotechnology volume 11, Article number: 531 (2009)
Abstract
Recently, deep sea water (DSW) has started to receive much attention for therapeutic intervention in some lifestyle diseases. In this study, the anti-obesity and antidiabetic effects of DSW in ob/ob mice were investigated. The animals were randomly divided into two groups with six animals: control group received tap water; the experimental group was treated with DSW of hardness 1000 for 84 days. The body weight gain after 84 days in DSW-fed group was decreased by 7% compared to the control group. The plasma glucose levels in the DSW-fed mice were substantially reduced by 35.4%, as compared to control mice. The results of oral glucose tolerance test revealed that DSW-fed groups significantly increased the glucose disposal after 84 days. DSW increased plasma protein levels of adiponectin and decreased plasma protein levels of resistin, RBP4, and fatty acid binding protein. Moreover, GLUT4 and AMP-activated protein kinase levels in skeletal muscle tissue were increased while peroxisome proliferator-activated receptor γ and adiponectin were decreased in adipose tissue of DSW-fed mice. These results suggest that the antidiabetic and anti-obesity activities of DSW were mediated by modulating the expression of diabetes- and obesity-specific molecules. Taken together, these results provide a possibility that continuous intake of DSW can ameliorate obesity and diabetes.
Introduction
Deep sea water (DSW) generally refers to sea water from a depth of more than 200 m in depth. DSW is characterized by purity, cold temperature, abundant nutrients, and minerals. Currently, DSW has been applied to food, agriculture, cosmetic, and medical field due to its high contents of unique minerals such as, magnesium (Mg), calcium (Ca), potassium (K), zinc (Zn), vanadium (V), etc. (Nakasone and Akeda 1999; Kimata et al. 2001, 2002; Hataguchi et al. 2005; Katsuda et al. 2008).
The recent life style and eating habits with increase of glucose and lipid intakes and lack of exercise caused a major problem of insufficient minerals. In order to replenish lacking minerals in body, various applications using mineral of DSW have been tried (Nakasone and Akeda 1999; Hataguchi et al. 2005). Although many types of health foods related to DSW are commercially available (e.g. mineral water, jelly, soy sauce, Japanese sake, confectioneries, salt, etc.), there are limited scientific proofs for its biological activities.
In our previous study (Hwang et al. 2008), it was found that DSW has inhibitory effects on adipocyte (3T3-L1) differentiation, suggesting a possibility for anti-obesity activity of DSW. We also found that an artificial mineral water containing only Ca and Mg showed also higher inhibitory effects (82% of original DSW) on adipocyte differentiation. This suggests that these two minerals played an important role in inhibitory effect of adipocyte differentiation.
Emerging research suggests that Ca and Mg intake may have a protective effect against obesity and diabetes (Robertson 2006; Sales and Pedrosa 2006). In this regard, it is worthy to study about the effects of DSW containing high content of Ca and Mg on antidiabetes and anti-obesity.
There are various diabetic animal models which can be used to investigate the pathogenesis and evolution of diabetes and can possibly be used to screen new antidiabetic drugs (Shafrir 1992). Animal models of type 2 diabetes are likely to be as complex and heterogeneous as the human condition. Some strains maintain euglycemia by mounting a robust and persistent compensatory β-cell response, matching the insulin resistance with hyperinsulinemia (Shafrir 1992; Rees and Alcolado 2005). The ob/ob mouse is a good model animal for studying both type 2 diabetes and obesity because it is unable to maintain the high levels of insulin secretion required throughout their lives and is able to maintain increased high body weight.
To date, to the best of our knowledge, there is no report describing the effect of DSW on diabetes, and there is only one publication on body weight gain. Katsuda et al. (2008) reported that DSW drinking did not affect reduction of body weight in Kusanagi-Hypercholesterolemic rabbits after feeding for 6 months. The objective of the present study was therefore to assess the effects of DSW on the lowering body weight and blood glucose levels in ob/ob mice.
Materials and Methods
Deep Sea Water
DSW, pumped up from a depth of 1.1 km and a distance of 18 km off Yangyang (128°56′ 42″ E, 38°00′44″ N, Gangwon-Do, Korea) by Watervis Co., Ltd. (Seoul, Korea), was filtered by micro-filter system (polytetrafluoroethylene, pore size 0.2 μm, Woongjin Chemical Co., Ltd., Seoul, Korea) to remove the phytoplankton and marine microorganisms. Filtered DSW was passed through the reverse osmotic membrane (Krosys, Seoul, Korea) and obtained the brine and the desalinated water. Next, we mixed the brine and the desalinated water to prepare the processed DSW having mineral ratio Mg/Ca/K/Na = 3:1:1:1. Generally, the hardness is defined as characteristic of water that represents the total concentration of polyvalent ion such as Ca, Mg, Fe, Mn, and Zn expressed as calcium carbonate (Veríssimo et al. 2007). The preferred method for determining hardness is to compute it from the results of separate determinations of Ca and Mg. In this study, we defined the hardness of DSW focusing on the concentration of Ca and Mg ions. The hardness values were calculated according to the following equation (Standard methods for the examination of water and wastewater 2-37 (1998):Hardness=Mg(mg/L)×4.1+Ca(mg/L)×2.5.Hardness=Mg(mg/mgLL)×4.1+Ca(mg/mgLL)×2.5.
Table 1 shows mineral composition of tap water and processed DSW of hardness 4000 used in this study. Elemental analyses of DSW were performed using inductively coupled plasma atomic emission spectroscopy (IRIS Intrepid II Duo, Thermo Fisher Scientific Inc., Waltham, MA, USA). The emission intensity measurements were made under the following conditions: RF Power 1,350 W, nebulizer flow 25 psi, and auxiliary gas 0.5 L/min. The calibration graph for each element was built with solutions prepared from 1 mg/mL stock solution (SCP SCIENCE, Ltd., Quebec, Canada). Determination of nonmetals such as Cl and SO4 were analyzed by ion chromatography (861 Advanced compact, Metrohm AG, Basel, Switzerland) under the following conditions: column, A Supp5 150; flow rate, 0.7 mL/min; pressure, 7.3 MPa; temperature, 20°C.Table 1 Mineral contents of processed deep sea water and tap water used in this studyFull size table
Animals and Breeding Conditions
All experiments were performed on male C57BL/6J ob/ob mice purchased from Japan SLC, Inc. (Hamamatsu, Japan) and left to acclimatize for 2 weeks before the experimental period (84 days). The animal breeding room was maintained under a constant 12-h light/12-h dark cycle with temperature 23 ± 2°C and relative humidity 55 ± 5% throughout the experimental period. They were given free access to standard pellets (Samyang Co., Seoul, Korea) and either DSW or tap water (Table 1). These experiments were approved by the Committee for Laboratory Animal Care and Use, Daegu University. All procedures were conducted in accordance with the “Guide for the Care and Use of Laboratory Animals” published by the National Institutes of Health.
Experimental Design
All the animals were randomly divided into two groups with six animals in each group: the control group received tap water; the experimental groups were treated with processed DSW (hardness 1000) for 84 days. Fasting blood glucose levels and body weight were measured after fasting the animals for 4 h (starting from 10:00 A.M.). Blood glucose levels were determined from the tail vein blood samples at 2:00 P.M. using a Glucose Analyzer (Lifescan, Milpitas, CA, USA).
Oral Glucose Tolerance Test
Oral glucose tolerance test (OGTT) was performed on day 84, by fasting the mice for 15 h, followed by an oral administration of glucose (2 g glucose/kg body weight). Blood glucose levels were determined from whole blood samples from the tail vein at −30, 0 (prior to glucose administration), 30, 60, 120, and 180 min after glucose administration.
Analytical Methods
The body weight gain and food intake were periodically measured. Blood samples of the experimental animals were collected in tubes treated with 0.1 M ethylenediamine tetraacetic acid as anticoagulant, and plasma was separated by centrifugation at 3,000×g for 10 min. The total cholesterol and triglyceride levels were measured using an enzymatic colorimetric assay (YD Diagnostics Co., Ltd., Seoul, Korea). Differences after OGTT were also analyzed with respect to each time point adjusted area under the curve (ΔAUC). A result of a test was statistically significant if the P value was less than 0.05.
Immunoblot Analysis
The levels of the adipogenesis-specific proteins of interest were analyzed by Western blot analysis as described below. An aliquot of plasma (100 μg) was diluted in 2× sample buffer [50 mM Tris (pH 6.8), 2% sodium dodecyl sulfate (SDS), 10% glycerol, 0.1% bromophenol blue, and 5% β-mercaptoethanol] and heated for 5 min at 95°C before SDS-polyacrylamide gel electrophoresis (PAGE) gel (7.5% and 12%). Subsequently, they were transferred to a polyvinylidene fluoride (PVDF) membrane and incubated overnight with 5% blocking reagent (Amersham Biosciences) in Tris-buffered salt (TBS) containing 0.1% Tween 20 at 4°C. The membrane was rinsed in four changes of TBS with Tween 20 (10 mM Tris–HCl, 150 mM NaCl, 0.1% Tween 20, pH 8.0), incubated twice for 5 min and twice for 10 min in fresh washing buffer and then incubated for 2 h with blocking solution containing 1:200 dilution of primary antibody (anti-goat fatty acid binding protein (FABP), Santa Cruz Biotechnology, Santa Cruz, CA, USA; anti-mouse RBP4, ABNOVA Co, Taipei, Taiwan; anti-mouse Resistin, R&D Systems, Minneapolis, MN, USA; anti-mouse adiponectin, BioVision, Mountain View, CA, USA). After four washes, the membrane was incubated for 2 h with horseradish peroxidase-conjugated anti-goat IgG, anti-mouse IgG, and anti-rabbit IgG secondary antibody (1:1,000, Santa Cruz Biotechnology) and developed using enhanced chemiluminescence (Amersham Biosciences). The proteins from skeletal muscle and adipose tissues (100 μg each) were isolated immediately after sacrificing the mice (on day 84) and diluted in 2× sample buffer (50 mM Tris (pH 6.8), 2% SDS, 10% glycerol, 0.1% bromophenol blue, and 5% β-mercaptoethanol) and heated for 5 min at 95°C before SDS-PAGE gel (12%). Subsequently, they were transferred to a PVDF in the same method described above, in which primary antibodies used were as follows: anti-rabbit p-AMP-activated protein kinase (AMPK), Santa Cruz Biotechnology, Santa Cruz, CA, USA; anti-mouse adiponectin, BioVision, Mountain View, CA, USA; anti-mouse peroxisome proliferator-activated receptor γ (PPARγ), Santa Cruz Biotechnology, Santa Cruz, CA, USA; and anti-rabbit GLUT4, Santa Cruz Biotechnology, Santa Cruz, CA, USA. The Western blot was analyzed by scanning with a UMAX PowerLook 1120 (Maxium Technologies, Akron, OH, USA) and digitalizing using an image analysis software (KODAK 1D, Eastman Kodak, Rochester, NY, USA).
Statistical Analysis
All experimental results were compared by one-way analysis of variance (ANOVA) using the Statistical Package of Social Science (SPSS, ver. 14.0) program, and the data were expressed as means ± SE. Group means were considered to be significantly different at P < 0.05, as determined by the technique of protective least significant difference when ANOVA indicated an overall significant treatment effect, P < 0.05.
Results
Effect of DSW on Body Weight Gain, Food and Water Intake
A total of 12 ob/ob mice were grouped into control (n = 6) and DSW-treated group (n = 6) having almost equal body weight and blood glucose levels. All mice had free access to either tap water or DSW during the entire experimental period (84 days). Obesity status was monitored by measuring diet intake and body weight because diet intake is generally greater in the obese state. Control group (tap water-fed mice) showed increased body weight gain which is one of the characteristic features of obesity. In contrast, a significant reduction in body weight gain was clearly observed from the first week of experiments as shown in Fig. 1. The final mean body weight of DSW-treated mice was decreased by about 7% compared to control mice. These results indicate that DSW is effective in reducing body weight gain (P < 0.05). There was no significant difference in food and water intake between the control and DSW-treated mice (Figs. 2 and 3), suggesting that body weight reduction in DSW-fed mice was not a consequence of reduced food intake.
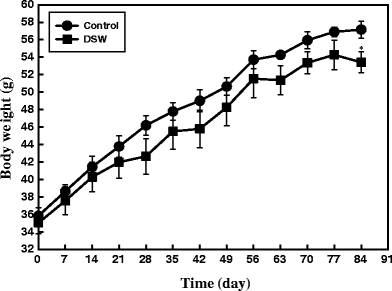
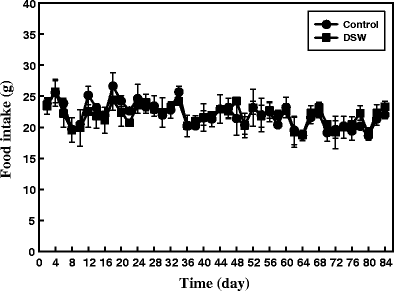
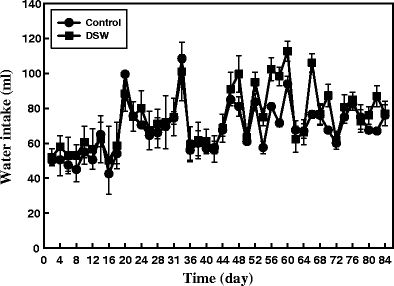
Effect of the DSW on Fasting Blood Glucose Levels
Normally ob/ob mice, in addition to increased body weight, also exhibit chronic hyperglycemia, a characteristic feature of diabetes. In our study, control group had elevated blood glucose levels while the treated group showed its attenuation during the course of DSW treatment, which was statistically significant at P < 0.01 on 84th day onwards (Fig. 4). This trend was almost constant till the end of experiment. As shown in Fig. 4, all ob/ob mice had high blood glucose levels from the starting day to day 35, and thereafter the levels were slowly decreased for both groups. Overall, the blood glucose levels in ob/ob mice were lower than those in control mice. When treated with DSW, the final fasting blood glucose levels decreased by 35.4% after 84 days, compared to the control mice (P < 0.01).
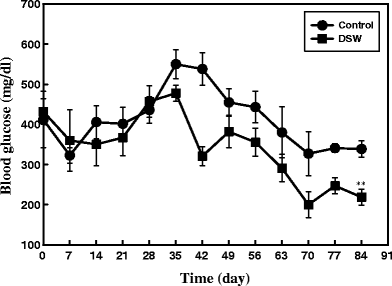
Effects of DSW on the Oral Glucose Tolerance Test
After 84 days of DSW treatments, glucose tolerance was examined for the two experimental groups using the OGTT methodology. Figure 5 shows the changes in the levels of blood glucose and ΔAUC during OGTT (2 g glucose/kg body weight). Compared with control mice, DSW-treated mice showed a remarkable improvement in overall glucose response. Although all groups showed a significant increase in the blood glucose at 30 min, the glucose levels in DSW-treated mice returned approximately to baseline after 120 min. In the course of OGTT, ΔAUC blood glucose response was also reduced by about 14% (P < 0.05) as compared to control mice (inside panel of Fig. 5). Taken together, these results clearly suggest the ability of DSW to reduce blood glucose levels and to increase glucose tolerance.
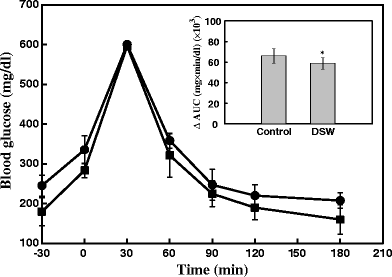
Effect of DSW on Plasma Lipids
The effects of DSW on plasma lipid concentration were studied after oral administration for 84 days. As shown in Fig. 6, the total triglyceride levels were decreased in DSW-fed mice (P < 0.05, P < 0.01) compared to control mice.
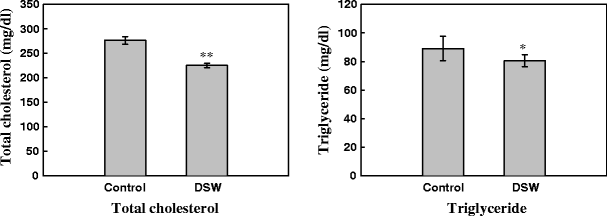
Effect of DSW on Adipogenic Proteins in Plasma
To identify differentially expressed proteins in response to DSW treatment, the immunoblottings were conducted for four proteins (adiponectin, FABP, RBP4, and resistin) which are well known for obesity disorder. The plasma level of adiponectin was increased, and those of other three proteins were decreased in response to DSW treatment, which were statistically significant (P < 0.05, P < 0.01) when compared to control (Fig. 7).
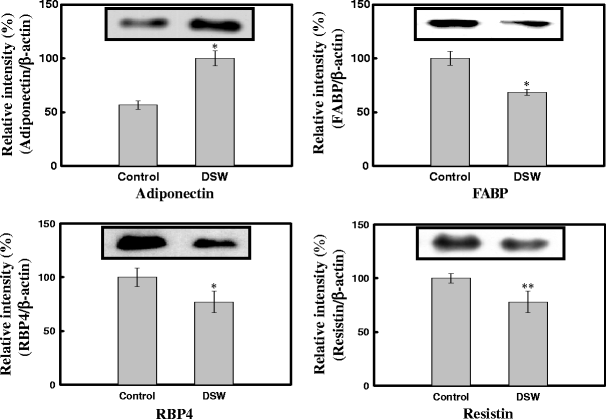
Effect of DSW on Expressions of Adipogenic Proteins in Adipocyte Tissue
Differential expression of the three important proteins (adiponectin, PPARγ, and AMPK) involved in adipogenesis event in adipose tissue between control and DSW-treated mice were analyzed by Western blotting. As shown in Fig. 8, DSW treatment decreased the protein levels of adiponectin and PPARγ, while it increased the levels of AMPK (active phospholylated form).
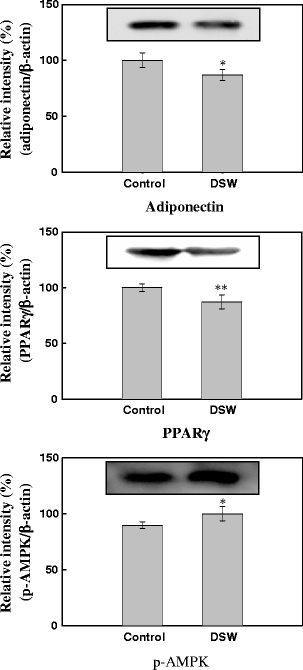
Effect of DSW on Adipogenic Proteins in Muscle
Figure 9 shows the differential plasma levels of AMPK and GLUT 4 in control and DSW-fed mice. The protein levels of both AMPK and GLUT4 were elevated in response to DSW treatment.
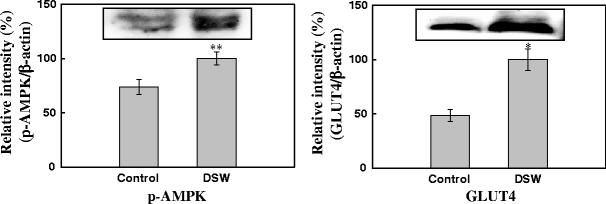
Discussion
In the present study, we addressed the anti-obesity and antidiabetic potentials of DSW. To date, there have been few studies on application of DSW to biotechnological fields (Matsubayashi et al. 1994; Fukami et al. 1997). Recently, some scientific evidences of therapeutic and preventive effects of DSW have been provided by Japanese research groups, which include alleviation of hyperlipidermia, atherosclerosis, hypertension, atopic eczema/dermatitis syndrome, and allergic skin responses (Kimata et al. 2001, 2002; Ueshima et al. 2003; Yoshioka et al. 2003; Miyamura et al. 2004; Hataguchi et al. 2005; Katsuda et al. 2008). In particular, DSW was effective in the prevention of hyperlipidemia and atherosclerosis in cholesterol-fed animals. A possible mechanism for this effect has been proposed: increased glutathione peroxidase activity and suppressive absorption of cholesterol in the small intestine played roles in these effects (Yoshioka et al. 2003; Miyamura et al. 2004; Katsuda et al. 2008).
Among many kind of minerals, Ca and Mg were proved to play pivotal roles in inhibitory effect on adipocyte differentiation (Hwang et al. 2008). The role of Ca in mediating multiple cellular functions has well been established by many investigators (Zemel 1998; Worrall and Olefsky 2002). More importantly, intracellular Ca acts as a second messenger in many signal transduction pathway. It has become clear that oral Ca supplementation could improve cellular insulin metabolism and partially correct insulin resistance in both animal and human patients (Choi et al. 2005; Barba and Russo 2006). Insulin-mediated glucose transport is affected by increasing the intracellular levels of Ca (Draznin et al. 1987). Recent human and animal studies indicated that a higher Ca intake was associated with reduced body fat or less gain of body fat over time, and possible mechanisms of intracellular Ca for fat metabolism have been demonstrated by Zemel and his group (Zemel 1998; Zemel et al. 2000; Xue et al. 2001). These studies showed that high dietary Ca intake is associated with reduced 1,25-vitamin D levels which in turn act in decreasing Ca influx into the cell and, thus, the intracellular levels of the ion: these modifications eventually stimulate lipolysis and inhibit lipogenesis in the adipocyte. In contrast, high intracellular Ca levels (low dietary Ca) are associated with increased fat synthesis and reduced lipolysis via Ca-dependent mechanisms. Dietary Ca could influence fat metabolism not only by modulating it within the adipocyte but also by increasing fecal fat excretion, as has been shown during a high Ca diet (Jacobsen et al. 2003; Papakonstantinou et al. 2004; Barba and Russo 2006). Intracellular Ca also modulates de novo lipogenesis in both rodent and human adipocytes, thereby representing a key therapeutic target for the control of obesity (Zemel 1998; Worrall and Olefsky 2002; Wagner et al. 2007). Increase of Ca in a variety of cells, including murine and human adipocytes, stimulates both the expression and activity of adipocyte fatty acids synthase and increase triglyceride accumulation in a Ca-dependent manner (Zemel 1998). The mechanism by which Ca might modulate body weight is unclear, but researchers hypothesize that increased intakes of dietary Ca result in decreased levels of intracellular Ca, triggering increased lipolysis (Zemel et al. 2000; Venti et al. 2005). As mentioned above, many studies have shown an inverse relationship between Ca intake and human body weight (Zemel 2004; Zemel and Miller 2004). However, many recent experiments have failed to find this relationship (Shapses et al. 2004; Paradis and Cabanac 2005; Venti et al. 2005; Duengler et al. 2006; Wagner et al. 2007).
Mg affects many cellular functions, including transport of potassium and Ca ions, and modulates signal transduction, energy metabolism, and cell proliferation (Corica et al. 1999; Saris et al. 2000). There is an increasing interest in the role of intracellular Mg in preventing and managing disorders such as hypertension, cardiovascular disease, and diabetes (Lopez-Ridaura et al. 2004). Several studies have shown that, among patients with diabetes mellitus, the frequency of hypomagnesemia is higher than expected and that it is correlated with the degree of severity of hyperglycemia (Saris et al. 2000). In type 2 diabetic patients, daily Mg administration, restoring a more appropriate intracellular Mg concentration, contributes to improve insulin-mediated glucose uptake. It has been shown that oral Mg supplementation improves the control of diabetes (Sjögren et al. 1988; Paolisso et al. 1992). A poor intracellular Mg concentration may result in a defective tyrosine-kinase activity at the insulin receptor level and exaggerated intracellular Ca concentration (Paolisso and Barbagallo 1997) because Mg concentration is critical in the phosphorylation of the tyrosine-kinase of the insulin receptor as well as all other protein-kinases. That is, the lower the basal Mg, the greater the amount of insulin required to metabolize the same glucose load, indicating decreased insulin sensitivity (Barbagallo and Dominguez 2007). Taken together, it is believed that a lack of magnesium leads to increased insulin resistance, and thus, low magnesium levels are an important risk factor for diabetes (Tosiello 1996). In this regards, DSW can be a beneficial agent to alleviate obese and/or diabetic status. Although Mg supplementation seems to have beneficial effect in diabetic patients, no rule about dosage and duration of the treatment with this mineral has been established until the moment (Sales and Pedrosa 2006). Therefore, for application of DSW to therapeutic purpose for diabetes in the future, more detailed guidelines are essentially required.
Many metabolic disorders such as obesity and diabetes are associated with dysregulation of adipocytokines (Zou and Shao 2008). For example, there are several reports with significant negative correlation between body mass index and plasma adiponectin levels (Arita et al. 1999; Cnop et al. 2003). Elevated plasma resistin levels were observed in the obese serum whose major sites of production in rodents are adipocytes while in humans, immunocompetent cells (Haluzik and Haluzikova 2006). In our results, a significant elevation of adiponectin and reduction in the plasma levels of resistin after DSW treatment implies increased insulin sensitivity. The elevated plasma RBP4 levels are also associated with metabolic syndromes such as obesity and type 2 diabetes (Graham et al. 2006; Gavi et al. 2007). Indeed, RBP4 augmented hepatic gluconeogenesis and attenuated insulin signaling in skeletal muscle. With this connection, our immunoblotting results clarified the effect of DSW on serum RBP4 attenuation, thus exerting its effect on insulin sensitivity and reduced glucose level.
AMPK provides an attractive target for therapeutic intervention in metabolic disorders such as obesity and type 2 diabetes. Indeed, experiments with animal models of type 2 diabetes and the metabolic syndrome show that activation of AMPK can reverse many of the metabolic defects in these animals in vivo (Iglesias et al. 2002). Coincidently, our results indicated that AMPK system could serve as the probable target of antidiabetic action of DSW.
It has been reported that the impairment of glucose uptake by adipose and muscle tissues in insulin resistance and obesity is associated with the reduction of cellular GLUT4 content (Zorzano et al. 1998; Huang and Czech 2007). Since the amelioration of abnormal glucose metabolism in type 2 diabetes is shown to be largely due to an increase in the glucose uptake in muscle and adipose tissues (Wing et al. 1994), the alteration in GLUT4 expression or in its translocation to the cellular membrane is regarded as a potential targets in the treatment of diabetes. In conclusion, it has been demonstrated that DSW might play beneficial roles in glucose metabolism in ob/ob mice via activation of AMPK and GLUT4 in skeletal muscle.
References
- Arita Y, Kihara S, Ouchi N, Takahashi M, Maeda K, Miyagawa J, Hotta K, Shimomura I, Nakamura T, Miyaoka K, Kuriyama H, Nishida M, Yamashita S, Okubo K, Matsubara K, Muraguchi M, Ohmoto Y, Funahashi T, Matsuzawa Y (1999) Paradoxical decrease of an adipose-specific protein, adiponectin, in obesity. Biochem Biophys Res Commun 257:79–83PubMed Article CAS Google Scholar
- Barba G, Russo P (2006) Dairy foods, dietary calcium and obesity: a short review of the evidence. Nutr Metab Cardiovasc Dis 16:445–451PubMed Article Google Scholar
- Barbagallo M, Dominguez LJ (2007) Magnesium metabolism in type 2 diabetes mellitus, metabolic syndrome and insulin resistance. Arch Biochem Biophys 458:40–47PubMed Article CAS Google Scholar
- Choi HK, Willett WC, Stampfer MJ, Rimm E, Hu FB (2005) Dairy consumption and risk of type 2 diabetes mellitus in men: a prospective study. Arch Intern Med 165:997–1003PubMed Article Google Scholar
- Cnop M, Havel PJ, Utzschneider KM, Carr DB, Sinha MK, Boyko EJ, Retzlaff BM, Knopp RH, Brunzell JD, Kahn SE (2003) Relationship of adiponectin to body fat distribution, insulin sensitivity and plasma lipoproteins: evidence for independent roles of age and sex. Diabetologia 46:459–469PubMed CAS Google Scholar
- Corica F, Allegra A, Lentile R, Buemi M, Corsonello A, Bonanzinga S, Macaione S, Ceruso D (1999) Changes in plasma, erythrocyte, and platelet magnesium levels in normotensive and hypertensive obese subjects during oral glucose tolerance test. Am J Hypertens 12:128–136PubMed Article CAS Google Scholar
- Draznin B, Sussman K, Kao M, Lewis D, Sherman N (1987) The existence of an optimal range of cytosolic free calcium for insulin-stimulated glucose transport in rat adipocytes. J Biol Chem 262:14385–14388PubMed CAS Google Scholar
- Duengler F, Torremocha F, Yameogo M, Marechaud R, Hadjadj S (2006) Effect of dietary calcium intake on weight gain in type 2 diabetic patients following initiation of insulin therapy. Diabetes Metab 32:358–363PubMed Article CAS Google Scholar
- Fukami K, Nishimura S, Ogusa M, Asada M, Nishijima T (1997) Continuous culture with deep seawater of a benthic food diatom Nitzschia sp. Hydrobiologia 358:245–249Article CAS Google Scholar
- Gavi S, Stuart LM, Kelly P, Melendez MM, Mynarcik DC, Gelato MC, McNurlan MA (2007) Retinol-binding protein 4 is associated with insulin resistance and body fat distribution in non obese subjects without type 2 diabetes. J Clin Endocrinol Metab 92:1886–1890PubMed Article CAS Google Scholar
- Graham TE, Yang Q, Bluher M, Hammarstedt A, Ciaraldi TP, Henry RR, Wason CJ, Oberbach A, Jansson PA, Smith U, Kahn BB (2006) Retinol-binding protein 4 and insulin resistance in lean, obese, and diabetic subjects. N Engl J Med 354:2552–2563PubMed Article CAS Google Scholar
- Haluzik M, Haluzikova D (2006) The role of resistin in obesity-induced insulin resistance. Curr Opin Investig 7:306–311CAS Google Scholar
- Hataguchi Y, Tai H, Nakajima H, Kimata H (2005) Drinking deep-sea water restores mineral imbalance in atopic eczema/dermatitis syndrome. Eur J Clin Nutr 59:1093–1096PubMed Article CAS Google Scholar
- Huang S, Czech MP (2007) The GLUT4 glucose transporter. Cell Metab 5:237–252PubMed Article CAS Google Scholar
- Hwang HS, Kim SH, Yoo YG, Chu YS, Shon YH, Nam KS, Yun JW (2008) Inhibitory effect of deep sea water on adipogenesis in 3T3-L1 adipocytes. Mar Biotechnol. In press
- Iglesias MA, Ye JM, Frangioudakis G, Saha AK, Tomas E, Ruderman NB, Cooney GJ, Kraegen EW (2002) AICAR administration causes an apparent enhancement of muscle and liver insulin action in insulin-resistant high-fat-fed rats. Diabetes 51:2886–2894PubMed Article CAS Google Scholar
- Jacobsen R, Lorenzen JK, Toubro S, Krog-Mikkelsen I, Astrup A (2003) Effect of short-term high dietary calcium intake on 24-h energy expenditure, fat oxidation, and fecal fat excretion. Int J Obes 29:292–301Article CAS Google Scholar
- Katsuda SI, Yasukawa T, Nakagawa K, Miyake M, Yamasaki M, Katahira K, Mohri M, Shimizu T, Hazama A (2008) Deep-sea water improves cardiovascular hemodynamics in Kurosawa and Kusanagi-Hypercholesterolemic (KHC) rabbits. Biol Pharm Bull 31:38–44PubMed Article CAS Google Scholar
- Kimata H, Tai H, Nakajima H (2001) Reduction of allergic skin responses and serum allergen-specific IgE and IgE-inducing cytokines by drinking deep-sea water in patients with allergic rhinitis. ORL Nova 11:302–303Google Scholar
- Kimata H, Tai H, Nakagawa K, Yokoyama Y, Nakajima H, Ikegami Y (2002) Improvement of skin symptoms and mineral imbalance by drinking deep sea water in patients with atopic eczema/dermatitis syndrome (AEDS). Acta Medica (Hradec Králové) 45:83–84Google Scholar
- Lopez-Ridaura R, Willett WC, Rimm EB, Liu S, Stampfer MJ, Manson JE, Hu FB (2004) Magnesium intake and risk of type 2 diabetes in men and women. Diabetes Care 27:134–140PubMed Article CAS Google Scholar
- Matsubayashi T, Maruyama I, Kido S, Ando Y, Nakashima T, Toyota T (1994) Effects of deep seawater on the growth of several species of marine micro-algae. J Appl Phycol 6:75–77Article Google Scholar
- Miyamura M, Yoshioka S, Hamada A, Takuma D, Yokota J, Kusunose M, Kyotani S, Kawakita H, Odani K, Tsutsui Y, Nishioka Y (2004) Difference between deep seawater and surface seawater in the preventive effect of atherosclerosis. Biol Pharm Bull 27:1784–1787PubMed Article CAS Google Scholar
- Nakasone T, Akeda S (1999) The application of deep sea water in Japan. Proc 28th UJNR Aquac Panel Symp, UJNR Technical Report No. 28
- Paolisso G, Barbagallo M (1997) Hypertension, diabetes mellitus, and insulin resistance. The role of intracellular magnesium. Am J Hypertens 10:346–355PubMed Article CAS Google Scholar
- Paolisso G, DiMaro G, Cozzolino D, Salvatore T, D’Amore A, Lama D, Varricchio M, D’Onofrio F (1992) Chronic magnesium administration enhances oxidative glucose metabolism in thiazide treated hypertensive patients. Am J Hypertens 5:681–686PubMed CAS Google Scholar
- Papakonstantinou E, Flatt WP, Huth PJ, Harris RB (2004) High dietary calcium reduces body fat content, digestibility of fat, and serum vitamin D in rats. Obes Res 11:387–394Article Google Scholar
- Paradis S, Cabanac M (2005) Calcium deficiency cannot induce obesity in rats. Physiol Behav 85:259–264PubMed Article CAS Google Scholar
- Rees DA, Alcolado JC (2005) Animal models of diabetes mellitus. Diabet Med 22:359–370PubMed Article CAS Google Scholar
- Robertson DS (2006) Magnesium or calcium hypophosphite could be a treatment for obesity in humans. Medical Hypotheses 66:439–440PubMed Article CAS Google Scholar
- Sales CH, Pedrosa LFC (2006) Magnesium and diabetes mellitus: their relation. Clin Nutr 25:554–562PubMed Article CAS Google Scholar
- Saris NEL, Mervaala E, Karppanen H, Khawaja JA, Lewenstam A (2000) Magnesium: an update on physiological, clinical and analytical aspects. Clinica Chimica Acta 294:1–26Article CAS Google Scholar
- Shafrir E (1992) Animal models of non-insulin-dependent diabetes. Diabetes Metab Rev 8:179–208PubMed CAS Article Google Scholar
- Shapses SA, Heshka S, Heymsfield SB (2004) Effect of calcium supplementation on weight and fat loss in women. J Clin Endocrinol Metab 89:632–637PubMed Article CAS Google Scholar
- Sjögren A, Floren E, Nilsson A (1988) Magnesium, potassium and zinc deficiency in subjects with Type II diabetes mellitus. Acta Med Scand 224:461–465PubMed Google Scholar
- Standard methods for the examination of water and wastewater 2-37 (1998) #2340 hardness. 20th ed. American Public Health Association/American Water Works Association/Water Environment Federation, Washington, DC, USA
- Tosiello L (1996) Hypomagnesemia and diabetes mellitus. A review of clinical implications. Arch Intern Med 156:1143–1148PubMed Article CAS Google Scholar
- Ueshima S, Fukao H, Okada K, Matsuo O (2003) Suppression of the release of type-1 plasminogen activator inhibitor from human vascular endothelial cells by Hawaii deep sea water. Pathophysiology 9:103–109PubMed Article CAS Google Scholar
- Venti CA, Tataranni PA, Salbe AD (2005) Lack of relationship between calcium intake and body size in an obesity-prone population. J Am Diet Assoc 105:1401–1407PubMed Article CAS Google Scholar
- Veríssimo MIS, Oliveira JA, Gomes MTS (2007) Determination of the total hardness in tap water using acoustic wave sensors. Sens Actuators B Chem 127:102–106Article CAS Google Scholar
- Wagner G, Kindrick S, Hertzler S, DiSilvestro RA (2007) Effects of various forms of calcium on body weight and bone turnover markers in women participating in a weight loss program. J Am Coll Nutr 26:456–461PubMed CAS Google Scholar
- Wing RR, Blair EH, Bononi P, Marcus MD, Watanabe R, Bergman RN (1994) Caloric restriction per se is a significant factor in improvements in glycemic control and insulin sensitivity during weight loss in obese NIDDM patients. Diabetes Care 17:30–36PubMed Article CAS Google Scholar
- Worrall DS, Olefsky JM (2002) The effects of intracellular calcium depletion on insulin signaling in 3T3-L1 adipocytes. Mol Endocrinol 16:378–389PubMed Article CAS Google Scholar
- Xue B, Greenberg AG, Kraemer FB, Zemel MB (2001) Mechanism of intracellular calcium ([Ca2+]i) inhibition of lipolysis in human adipocytes. FASEB J 15:2527–2529PubMed CAS Google Scholar
- Yoshioka S, Hamada A, Cui T, Yokota J, Yamamoto S, Kusunose M, Miyamura M, Kyotani S, Kaneda R, Tsutsui Y, Odani K, Odani I, Nishioka Y (2003) Pharmacological activity of deep-sea water: examination of hyperlipemia prevention and medical treatment effect. Biol Pharm Bull 26:1552–1559PubMed Article CAS Google Scholar
- Zemel MB (1998) Nutritional and endocrine modulation of intracellular calcium: implications in obesity, insulin resistance and hypertension. Mol Cell Biochem 188:129–136PubMed Article CAS Google Scholar
- Zemel MB (2004) Role of calcium and dairy products in energy partitioning and weight management. Am J Clin Nutr 79:907S–912SPubMed CAS Google Scholar
- Zemel MB, Miller SL (2004) Dietary calcium and dairy modulation of adiposity and obesity risk. Nutr Rev 62:125–131PubMed Article Google Scholar
- Zemel MB, Shi H, Greer B, DiRienzo D, Zemel PC (2000) Regulation of adiposity by dietary calcium. FASEB J 14:1132–1138PubMed CAS Google Scholar
- Zorzano A, Santalucia T, Palacín M, Gumà A, Camps M (1998) Searching for ways to upregulate GLUT4 glucose transporter expression in muscle. Gen Pharmac 31:705–713CAS Google Scholar
- Zou C, Shao J (2008) Role of adipocytokines in obesity-associated insulin resistance. J Nutr Biochem 19:277–286PubMed Article CAS Google Scholar
Acknowledgments
This research was supported by the grant from the Basic Research Program of the Korea Science & Engineering Foundation (R01-2008-000-10277-0) and by Ministry of Maritime Affairs and Fisheries of Korean Government.
Author information
Affiliations
- Department of Biotechnology, Daegu University, Kyungsan, Kyungbuk, 712-714, South KoreaHee Sun Hwang, Hyun Ah Kim, Sung Hak Lee & Jong Won Yun
Corresponding author
Correspondence to Jong Won Yun.
Rights and permissions
About this article
Cite this article
Hwang, H.S., Kim, H.A., Lee, S.H. et al. Anti-obesity and Antidiabetic Effects of Deep Sea Water on ob/ob Mice. Mar Biotechnol 11, 531 (2009). https://doi.org/10.1007/s10126-008-9171-0
- Received18 July 2008
- Accepted26 November 2008
- Published13 December 2008
- DOIhttps://doi.org/10.1007/s10126-008-9171-0